nkx3.2 mutant zebrafish accommodate jaw joint loss through a phenocopy of the head shapes of Paleozoic jawless fish
– What happens if a mutation disables an apparatus after 500 million years of evolutionary conservation? An experiment targeting zebrafish jaw joints provides new insights –
Miyashita, T., P. Baddam, J. Smeeton, A.P. Oel, et al. (2020)
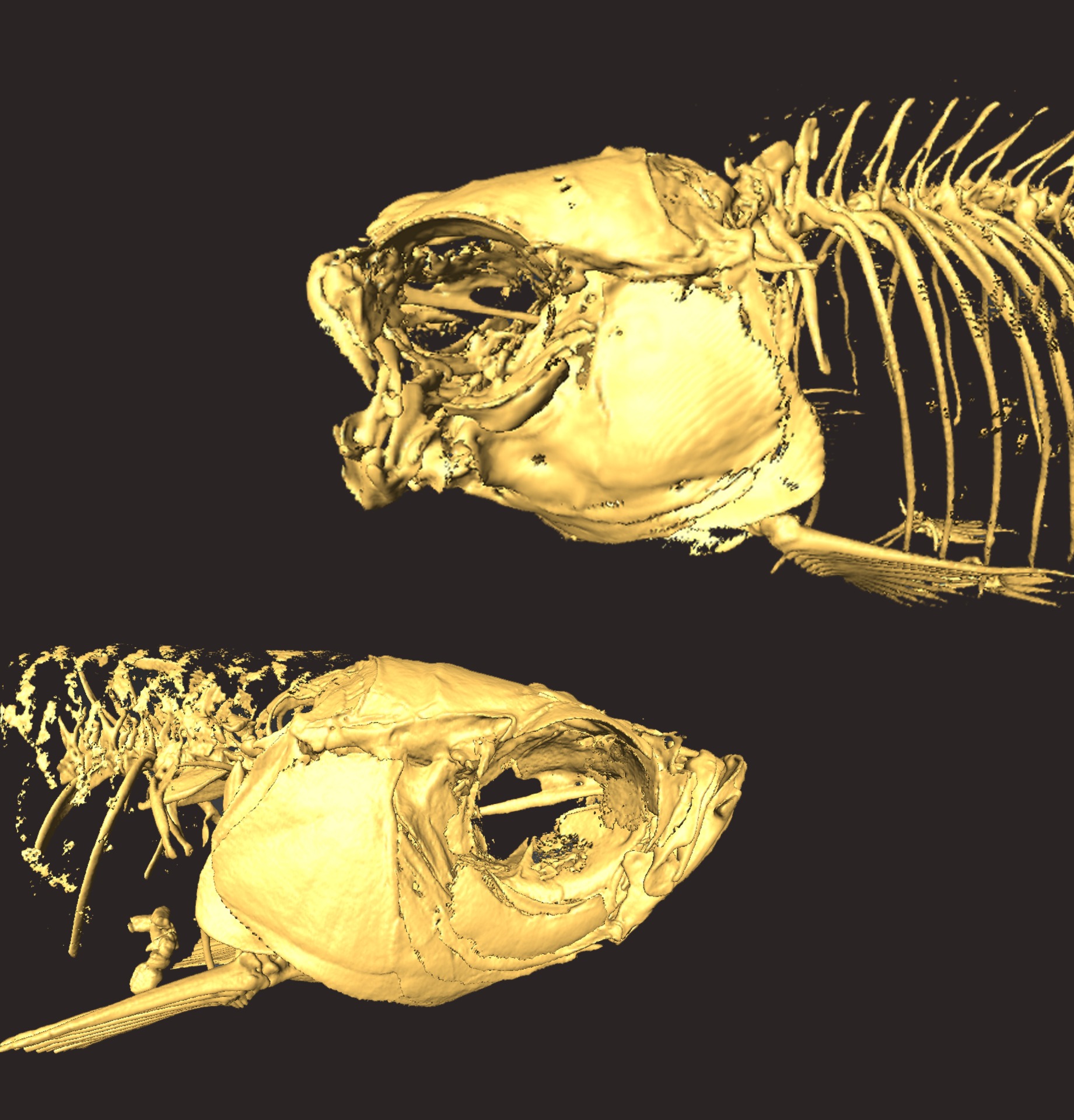
Highly modified skeletal anatomy of functionally jawless zebrafish. Top: skeleton of an adult nkx3.2 mutant zebrafish; Bottom: skeleton of an adult wildtype zebrafish.
From Miyashita et al. (2020)
Generating the fish that scream Edvard Munch
- The final step in the origin of vertebrate jaw is the acquisition of an hinge joint between upper and lower jaws. In non-mammalian jaw joints, Nkx3.2 plays a critical role in specification.
- In parallel with a project looking at Nkx3.2 expression and function in jawless lampreys, we engineered mutations in zebrafish nkx3.2.
- Two lines of nkx3.2-null mutants were generated. One harboring frameshift and 20 bp deletion in the homeodomain of nkx3.2; the other having a deletion across the start codon.
- Mutant zebrafish hatch in almost identical form with wildtype. However, the jaw joints are missing in these mutants. The upper and lower jaw cartilages are fused to one another at 4 dpf, and this condition persists through ontogeny.
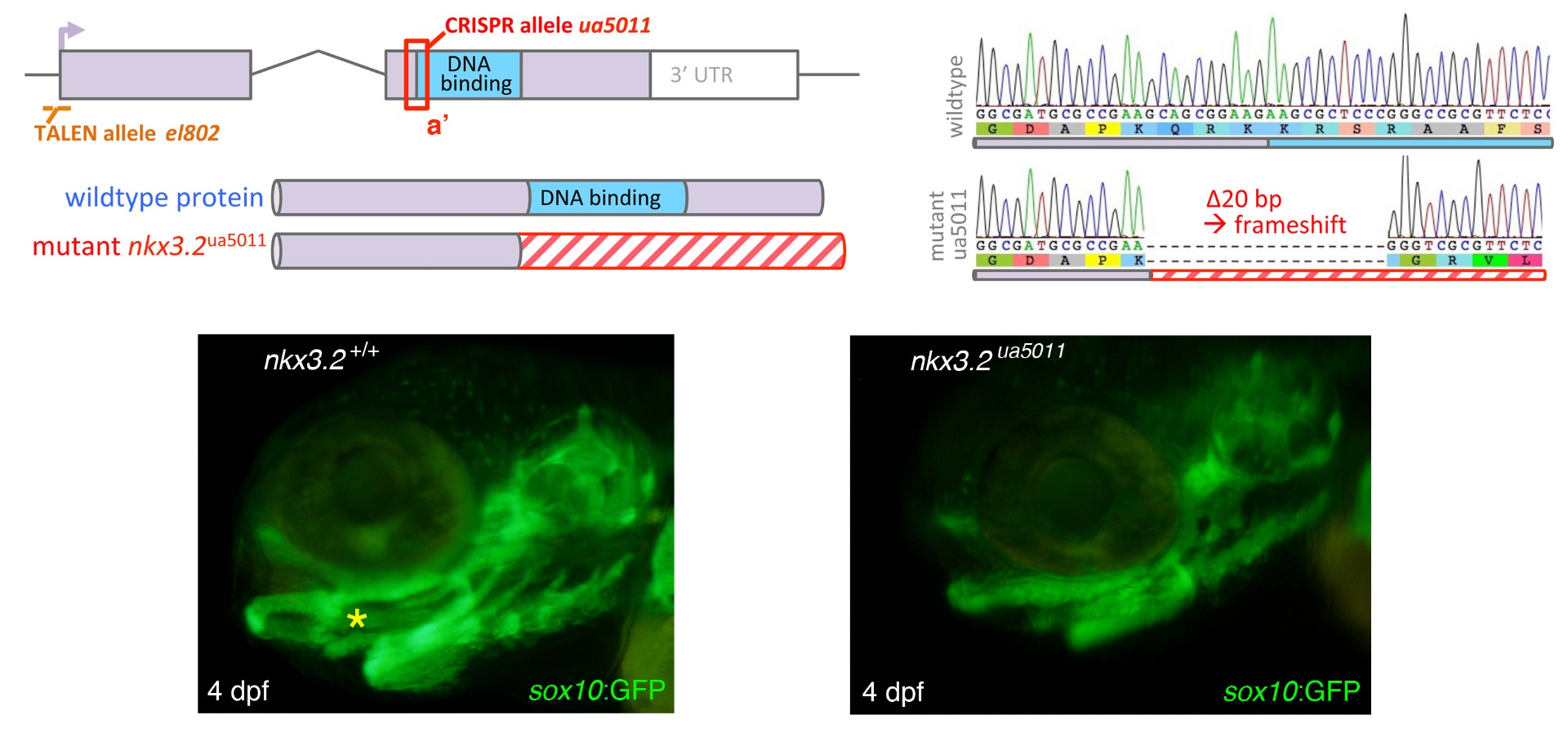
Top row: nkx3.2 mutation (ua5011) was engineered in the DNA-binding homeodomain of the gene, causing 20 bp deletion and a frameshift.
Bottom left: a 4-day old wildtype zebrafish with endogenous GFP expression by sox10 reporter, showing the cartilaginous skull. Yellow asterisk indicates the jaw joint.
Bottom right: a 4-day old nkx3.2 mutant zebrafish with endogenous GFP expression by sox10 reporter, showing the cartilaginous skull. The upper and lower jaw cartilages are fused into a single rod and the joint is absent.
From Miyashita et al. (2020)
- Marked shift appears when larval zebrafish start actively feeding, and prior to bones dominating in the skulls. From this point on, nkx3.2 mutants show a fixed large gape and permanently dropped jaws. The mutant phenotype continues to develop through the rest of the ontogeny, and the departure from that for wildtype progressively becomes larger.
- By the time they reach the adulthood, the nkx3.2 mutants have an open mouth phenotype: lower jaws depressed and fixed; premaxilla and maxilla abutted against the orbit and inmobile; snout highly reduced; downturned and hypertrophied basihyal; vertebral defects.
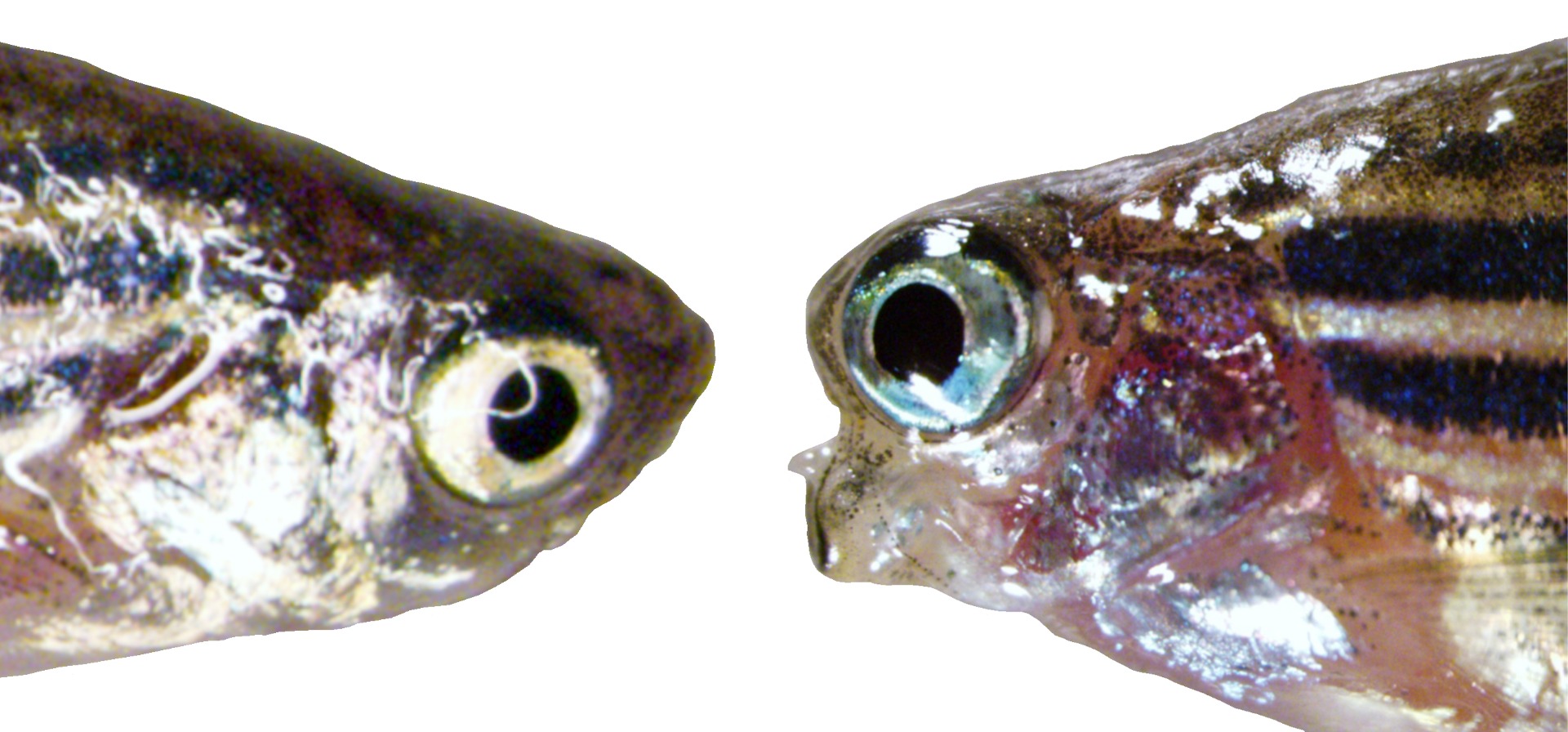
Left: 2-month old adult wildtype zebrafish; Right: 2-month old adult nkx3.2 mutant zebrafish, showing the open mouth phenotype.
From Miyashita et al. (2020)
How did we analyze the nkx3.2 mutant zebrafish?
- The initial effect of the mutation (fused jaw joints) is long lasting. The adult jaws remain non-mobile and fixed in the open mouth state.
- Curiously, the rest of this dramatic skull phenotype: a) develops long after the patterning role of nkx3.2 in skeletal development, but coincident with when the larvae flex their soft, mostly cartilaginous skull to feed, and becomes more pronounced as the bones develop to solidify the skull; and b) affects parts of the skull that are outside the expression domain of nkx3.2.
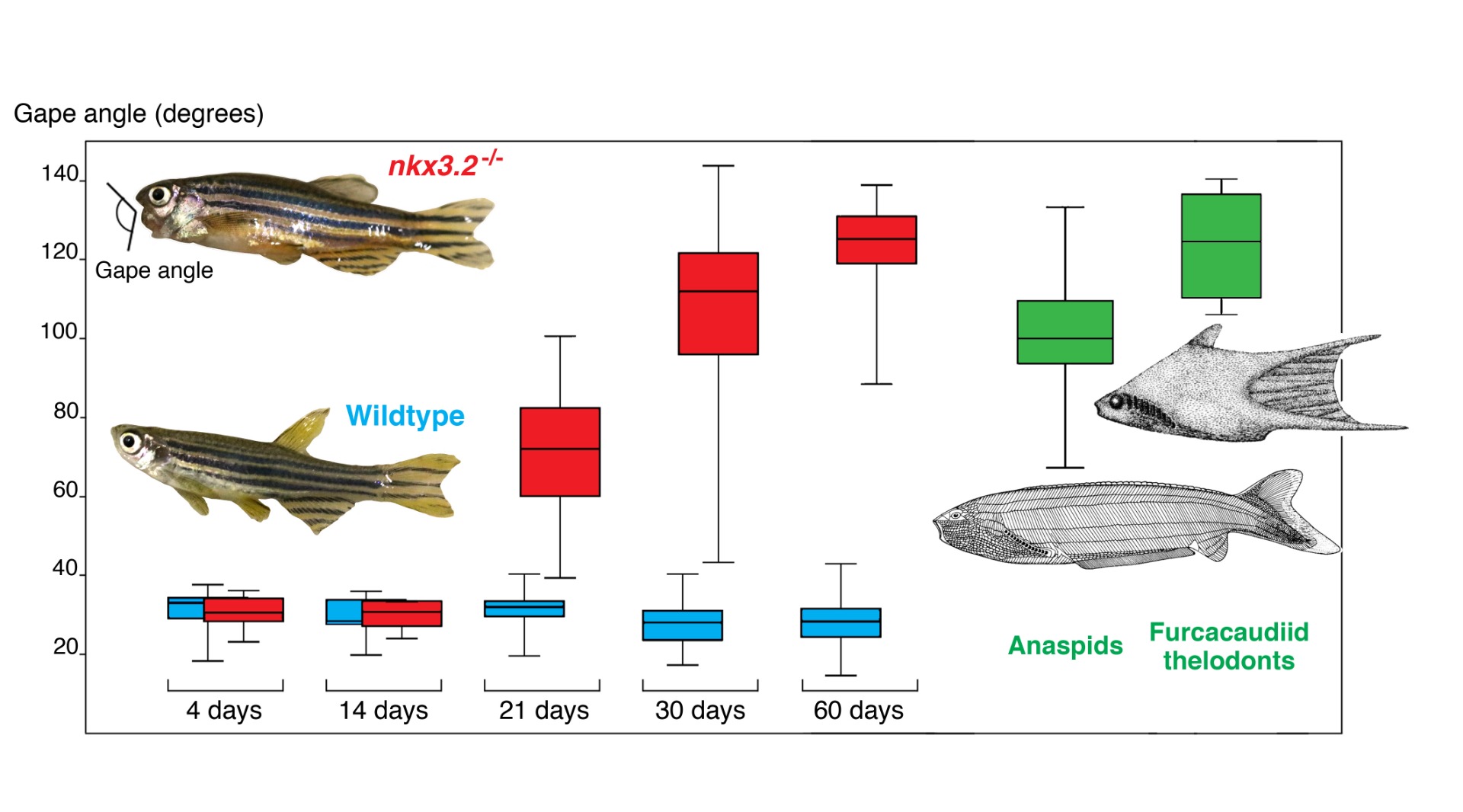
Gape angles across ontogenetic growth of zebrafish between wildtype (blue) and nkx3.2 mutants (red). The mutant gape angle begins to increase around the onset of active feeding and bones forming in the skull, and becomes wider toward the adulthood in which the jaws are fixed open widely, similar to the gape angles in extinct jawless fishes (green).
From Miyashita et al. (2020)
- This indicates that the dramatic open-mouth phenotype develops via developmental plasticity. Unable to move their jaws, the mutants could only feed with a permanently open mouth. To accommodate this, the mutant skulls are modified, not as a direct result of nkx3.2 mutation but responding to the jaw dysfunction caused by it.
- Surprisingly, the plastically altered skulls of nkx3.2 mutants resemble in overall proportions to the extinct jawless fishes called anaspids and furcacaudiform thelodonts. These 'agnathans' shared a similar functional condition (lacking a mobile jaw), and the overall body profile (laterally compressed, as in zebrafish).
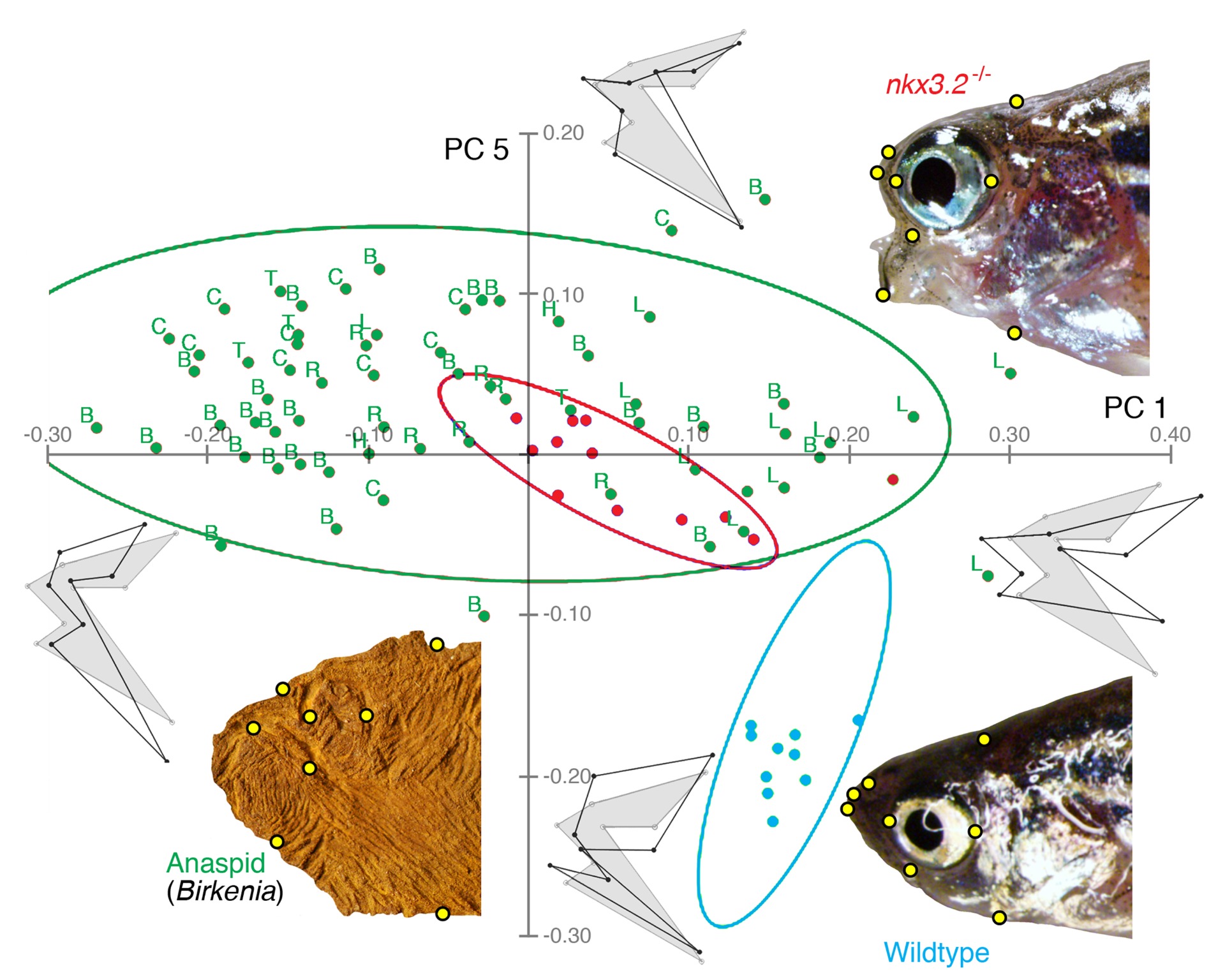
Comparison of head shapes among wildtype and nkx3.2 mutant zebrafish (adults) and anaspids (extinct jawless fishes). The adult mutants fall closer to anaspids than to wildtypes.
From Miyashita et al. (2020)
Do the jawless zebrafish mutants represent a reversal to ancestral jawless fish?
- No, and there are several reasons. 1) The fossil jawless fishes represent their own independent branches; they are not ancestors to zebrafish. 2) Despite similar head shapes, the skulls are made of different. 3) Nkx3.2 is a highly conserved gene, and was likely passed down in the fossil jawless fishes. Therefore, the nkx3.2-null mutants do not restore the same genomic state as these ancient fishes.
- Our mutants fall closer to the phenomenon known as convergence. Through different paths, both the mutant zebrafish and ancient jawless fishes acquired similarly shaped heads.

Similar head shapes among mutant zebrafish, anaspids, and thelodonts share no common ancestry, but represent independent derivation and convergence.
From Miyashita et al. (2020)
- What does this mean? Two things: 1) Even though an infinite number of possible combinations exists, vertebrates have limited repertoire to produce forms that make functional sense. 2) Development has latent potentials to generate such forms and accommodate mutation-driven defects. This adaptive nature of plasticity is widely known, but the case of nkx3.2 mutant zebrafish is extreme, showing how drastically skeletal shapes can be modified through plastic response.
- So, in that sense, these jawless mutant zebrafish provide an opportunity to learn about the enigmatic biology of fossil jawless fishes. Experiment is ongoing to look at how the mutants feed, and test whether this can be used as a partial model for feeding in the extinct jawless vertebrates.
Theoretical implications of functionally jawless zebrafish
- Vertebrates have a limited repertoire of skull configuration. Otherwise, functionally jawless zebrafish would be able to develop a variety of skull shapes to accommodate the jaw dysfunction, and would not converge onto agnathans so tightly. Therefore, this delineates constraints in vertebrate skull development with functional underpinnings.
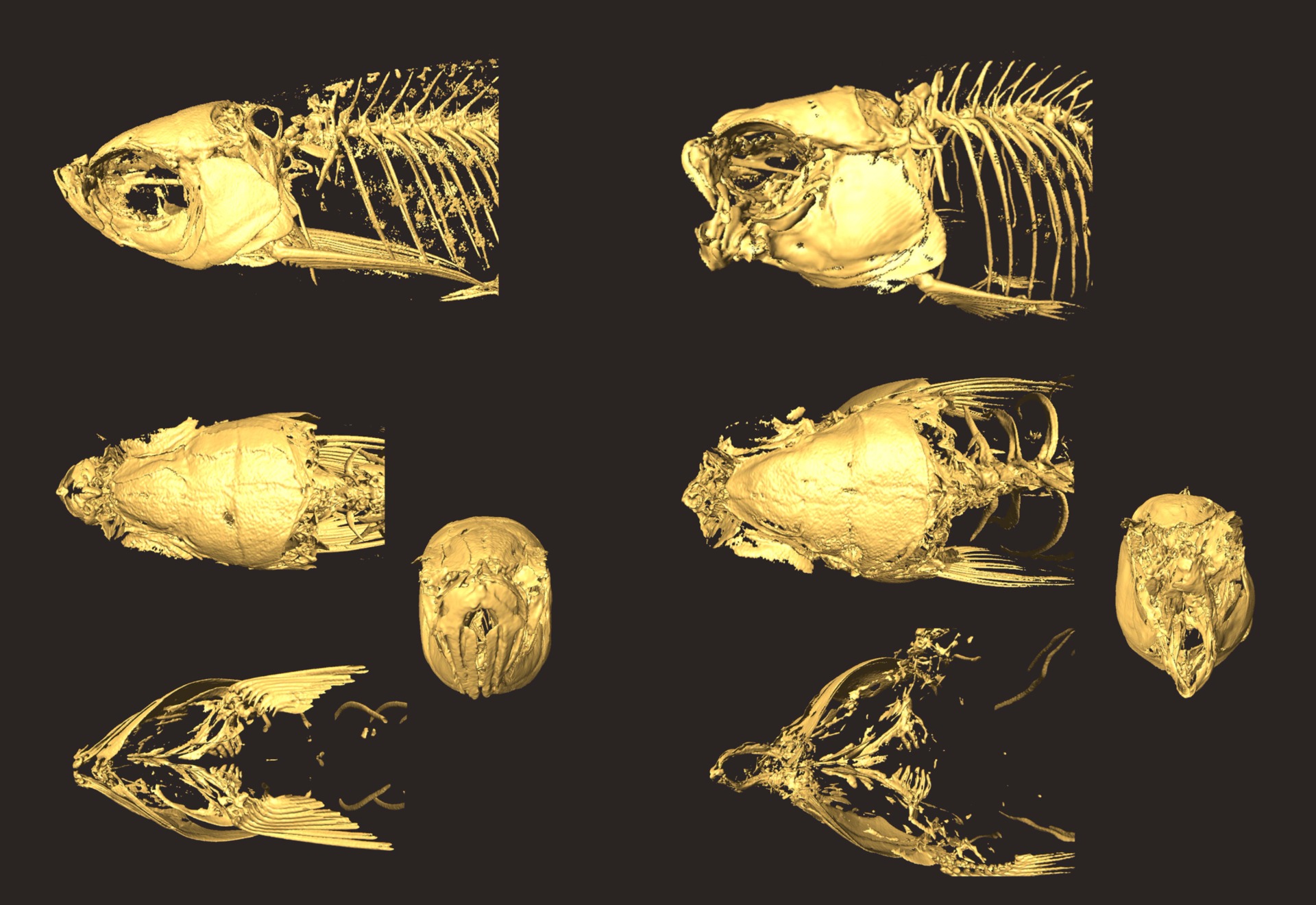
Multiple views of the wildtype (left) and nkx3.2 mutant (right) skulls. Reconstruction using µCT scan.
From Miyashita et al. (2020)
- Knockout or knockdown experiments sometimes produce phenotype that resembles outgroup (or perceived ancestral) condition in a living animal. This is typically interpreted as a phenocopy, and even used as inferences for the developmental mechanism behind evolutionary transitions (see some examples here, here, and here).
- But developmental plasticity – likes the one that caused the open-mouth phenotype in nkx3.2 mutant zebrafish – needs to be considered. Developmental process is fundamentally adaptive, and phenotypic accommodation can operate at any developmental stage (see remarkable examples here, here, and here; also, see a thoughtful review by Smith & Schneider).
Supplementary video accompanying Miyashita et al. (2020), showing the jaw morphology and feeding behaviors in the adult wildtype and nkx3.2 mutant zebrafish.
- Finally, the functionally jawless zebrafish present an interesting model with which to explore potential role of plasticity at major evolutionary transitions. Typically, mutations that affect an evolutionarily conserved structure are considered maladaptive. But their deleterious effects could be buffered through phenotypic accommodation coordinated by adaptive developmental plasticity. This is where I focus on in my ongoing research on nkx3.2 mutant zebrafish.
Resources
- Watch supplementary movie accompanying Miyashita et al. (2020), showing the jaw morphology and feeding behaviors of functionally jawless zebrafish.
- Read a follow-up study on the nkx3.2 mutant phenotype: Smeeton et al. (2021) Development 148: dev193409.
- Read more about phenotypic accommodation and developmental plasticity: West-Eberhart (2003, 2005a, 2005b); Palmer (2012).
- Media release from Canadian Museum of Nature
- Smeeton Lab at Columbia University
- Crump Lab at the University of Southern California
- Allison Lab at the University of Alberta
- Graf Lab at the University of Alberta